Why you need to know syntheses
Neo Chemica was a new employee at Acme Polymer Products. He was excited about his job, wanted to do well but really didn't know much about how polymers were made. His boss was of the old "sink or swim" school and had thrown Neo into the new synthesis group. Neo had been told to learn how to make polymers by solving problems.
He really thought it could't be all that hard. He'd aced all of his chemistry courses in college and loved doing organic synthesis. That had been the focus of his undergrad thesis. What could be hard about making polymers? They were just organic compounds of a slightly bigger nature.
He had been busy organizing his desk and lab space when his boss came in. "Neo, got a project for you. Well, two actually. Our biggest customer needs a couple of improvements in two of their polymer lines. Seems they've gotten orders for materials they don't actually have yet. Kind of "cart before the horse" behavior if you ask me. But for us? Not to question why, just do or die. Oh, and the "do or die" part is on you. Here, figure out how to make this happen." And with that, he passed Neo a description of what was needed and headed off to do important stuff of some sort...
Messing with Glass Transition and the Amorphous Phase
"Ok," Neo thought to himself, "Raising the Tg of one of their vinyl polymers shouldn't be too hard. Wonder what a Tg is anyway." To figure that out, what a Tg is, didn't take too long. He first asked some of his co-workers, who seemed amused at his lack of basic polymer knowledge. They sent him to the main reference site they used where he found out why polymers have a Tg and what an amorphous phase is. He ran across the picture below that's an analogy of the amorphous phase using spaghetti.
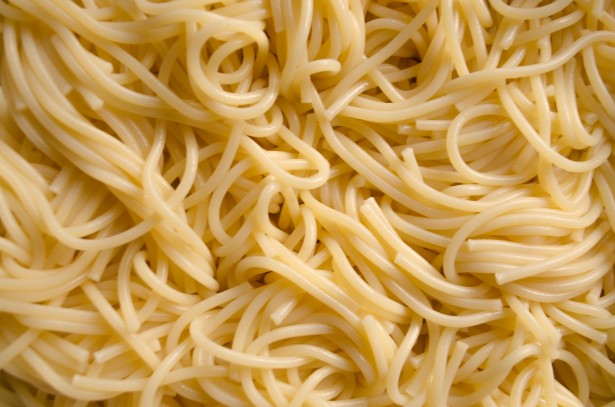
"Weird," he said on discovering how this unique behavior related to molecular composition. "Organic compounds I've worked with don't have that property. Ok, then, maybe polymers are a little different from what I'm used to. No biggie."
He knew the polymer he had to modify was a vinyl polymer. Easy to make although you had to do it without any oxygen present. While breathing is good for humans, it's not so good for radical propagation. Termination and side-reactions kill the polymer before it really gets going. For practice, he made a batch of the basic polymer. Turned out pretty well, although his lab mates gave him a hard time about conversion.
Next he looked at some of the other polymers they had in stock. Yeah, here's one that has a much higher Tg. Costs a lot more so the customer wasn't interested in buying it to cover their order. Well, maybe he could add some of the monomer for that polymer to the one that he had just used. Mix in a little pixie dust and the Tg should go up just enough...
Neo worked on this for a couple of days, figuring out all the requirements for monomer purity- much higher than he was used to for simple organics- and reactor atmosphere control- any oxygen at all and wham! no polymer. Finally got it all worked out and made a batch of copolymer that actually looked pretty good. So when analytical sent back a value for the Tg that was exactly what their customer needed, Neo was ecstatic. So was his boss and the customer. "Swim" was the operative word now for Neo.
His boss still thought the "sink or swim" approach was really the way to go or maybe he was thinking about his next promotion or game of golf, whatever. In any event, the pilot plant operator cranked out a large batch of material to send on for customer evaluation. And Neo was sent back to the lab to tackle problem number two: higher melting point for one of their crystalline polymers.
Why Crystallinity Isn't The Same In Polymers
And that concept had him really puzzled. He was used to crystalline organics- he'd been a whiz at getting the new compounds he made to crystallize to pure form. And those crystals he got were gorgeous, shimmery in the light and with such perfect regular structures. Seems most polymers didn't crystallize that way. They had "domains" of crystallinity but those were surrounded by a sea of amorphous regions, and of course, those had a Tg but no melting point. Most crystalline polymers weren't all that crystalline, being just semi-crystalline at less than 50%. A phrase from a movie he'd seen in college kept coming to mind: "It's complicated." Yeah, guess polymers are, he thought.
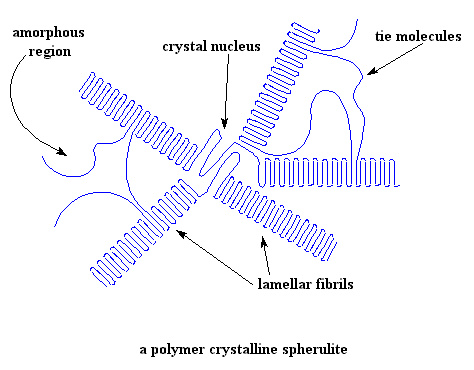
This project was more challenging than the last one but he finally decided to use the same approach. If throwing in a comonomer gave a higher Tg polymer then, he'd just add some monomer from a higher Tm polymer to the one he had to use here. He looked at their list of polymer products and picked one that had a really high Tm. He didn't pay too much attention to how it was made; in fact, didn't even bother to find out. "Well, hey," he thought to himself, "it's got a vinyl group like the one he'd already worked on. Vinyl's should all polymerize the same way."
He was beginning to think he'd really gotten this oxygen-free radical polymerization down pretty well at this point but then he opened the reactor on his newest attempt. To his surprise, the "polymer" he'd hoped to make was actually a gooey mess of sticky slop. If it had a Tm of some kind, it must be way below room temperature. Well, better find out what he'd made.
The chemist in analytical was smirking when he gave Neo the results. Seems he'd made a whole batch of really low molecular weight oligomers that would have to be disposed of somehow. And that cost money, something the boss didn't like to waste.
Neo tried again, same result. Now things were getting out of control. The boss finally put Neo with one of the more experienced lab personnel. "Nope, that monomer doesn't polymerize radically. It's a vinyl ether and only makes polymer with cationic initiation." Neo was totally flummoxed. He didn't even know what that process involved, let alone how to make it work here. In fact, the boss was just about fed up with Neo wasting money. Sure, he'd won on his first challenge but it was clear he just didn't know enough about polymers to work here. And he told Neo that, as he led him out of the lab and through the front door. "And don't come back till you know more about polymers!" Another budding career down the drain. Well, maybe there's hope for people like Neo. Somewhere, somehow...
Back to the Basics
So what's the point of this long-winded discourse? Did Neo ever learn what he needed to work in the polymer industry? Are all plastics made the same way? Does the moon rise and set on a regular basis? Does a bear...? Well, anyway, such imponderable questions won't help Neo, but for you, the interested student of polymer science, these and other questions will get you on the right track.
Okay, then, let's jump into what kinds of polymerizations there are and how they actually work to make high molecular weight polymers. For starters, let's reaffirm how we classify polymer synthetic methods. There are three basic ways to make polymers synthetically. Actually, there are more, but it helps to simplify things at the beginning. And by the way, nature has it's own methods which we'll touch on occasionally. Problem is, nature uses methods we don't fully understand and usually can't imitate effectively. Wish we could- nature makes some fantastic polymers like cellulose and spider silk that have outstanding properties. Oh, well, back to synthetics.
Traditional Definitions of Polymerization Types
Now, then, I'm sure you're just dying to find out what those three basic methods are. In a nutshell, they include:
=> Polycondensation or Step-growth
=> Polyaddition or Chain-growth
=> Ring-opening or Mixed-mode
Let's take a quick look at their differences and then dive into the details in the sections that follow. And for an alternative discussion of the first two of these, try this page on our sister site, "The Macrogalleria."
Traditional classifications are based on a simple macroscopic property: does the polymerization reaction give off some kind of small molecule that could be called a condensate? This is pretty broad and ill-defined. For example, the "small molecule" could be water vapor or some kind of gas like CO2 or N2 given off. And believe it or not, there are polycondensation reactions that do just that. These aren't really condensates at typical room temperatures and pressures but if you lower the temperature far enough, they will be. That would mean collecting a liquid nitrogen "condensate," for example, something that requires extremely low temperatures.
Oh, well, the key requirement is this: the chemical formula of the repeat unit is NOT the same as the combined formula of the monomers. Easy enough, but tells you absolutely nothing about the mechanism. As I said, this is a macroscopic definition- something you can (theoretically) see under normal atmospheric conditions by just looking.
The definition actually stems from the observation early in the development of polymers of condensate water that is split off during formation of ester groups or amide units during polyesterification or polyamidation. Water, of course, is easy to see collecting above or on the top of the reaction vessel. And methanol is another condensate formed during polycondensation reactions. See the figure below for the first step in the commercial process for making polyester [poly(ethylene terephthalate) or PET] by polycondensation. So far, so good.
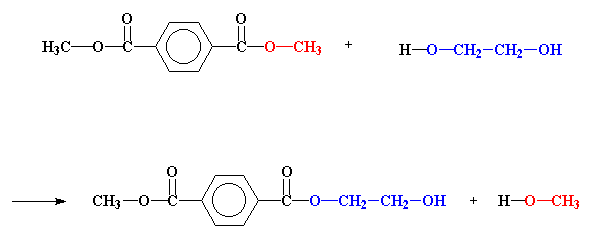
And I'm sure you've jumped ahead to the definition of a "polyaddition" polymerization. In this case, no condensate and the molecular formula of the repeat unit is exactly the same as the monomer or monomers.
That doesn't mean the repeat unit has exactly the same functional groups or organization of atoms. In fact, it doesn't and can't- the monomer has to "do something" that changes it to make it join up with other monomer units. Remember the train analogy in the "Introductory" course? The individual cars have to join together to make a train, and that involves the coupling "knuckle" to close and clamp onto the knuckle of the next car to make a connection that can survive being pulled on for hundreds of miles. The example below is of the overall reaction for making low-density polyethylene...
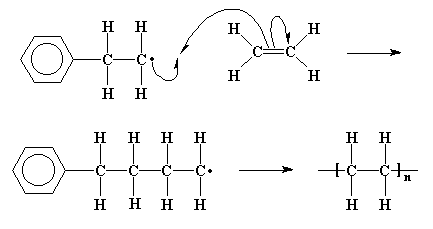
Okay, then, polycondensation involves forming a condensate during polymer formation while polyaddition doesn't. What about ring-opening? Is it one of these two or something different? If you read the list above carefully, you saw the term "mixed mode" ascribed to ring-opening polymerization. Simply put, that means the process usually has characteristics of both condensation and addition polymerizations. For example, in the conversion of caprolactone to polycaprolactone (see below), the polymer formed has an ester group in each repeat unit, similar to the ester groups in PET above. So in that sense, it looks like a polycondensation polymer. But hold your horses (assuming you have any)! There's no condensate formed because the cyclic monomer has exactly the same ester group. And that makes the conversion of monomer to polymer look more like a polyaddition reaction: same molecular composition of monomer and repeat unit.
Initiation Step for Ring-Opening Polymerization of Caprolactone

Propagation Step for Ring-Opening Polymerization of Caprolactone

Well, then, which is it? And the answer is why we call it "mixed mode:" the mechanism is just like polyesterification (a polycondensation reaction) involving a tetrahedral intermediate, but the kinetics are more like those of polyaddition reactions, fast and furious with polymer formed early on and throughout the polymerization. Oops, let the cat out of the bag in a way (what cat? what bag?). But we'll get to that later when we delve into the details of mechanisms for each of these types of polymerizations. Hope that whets your appetite for more (although "whetting" is usually ascribed to the process of sharpening a dull knife or axe, for what that's worth).
Mechanism Based Definitions for the three main types
Step-Growth Mechanism
Not to be too mysterious about the mechanism based definitions, but they are newer and less traditional. On the other hand, they're more related to the actual mechanism by which these types of polymers are made. So here you go:
The name "step-growth" implies something important about the process. The polymer grows "one step at a time." Specifically, that means the active chain ends (and usually both ends are reactive for this type) take on another reactive group one at a time. Chains grow slowly but monomers disappear early in the polymerization. Because both ends of any monomer, oligomer or polymer are reactive, ANY of these can react with any of the others: monomers with dimers, trimers and oligomers; monomers with any polymer; oligomers with oligomers; oligomers with polymer; and polymer with polymer.
Consequences of this behavior are that polymer molecular weight grows slowly and only gets to usable high molecular weight when monomers and oligomers are all converted to polymer, near the end of the polymerization. In addition, high molecular weight ONLY occurs when virtually all active chain ends are used up; that is, when conversion is approaching 100%. Here conversion refers to reactive groups and not the chains they're tied to. And next, which should be obvious, chains grow slowly and all together, no matter how small or big they are. See the graph below to visualize what I've said above. Look at the plot labeled "step growth," but you surely won't stop there, so see if you can figure out where we're going with the other two types given.

Image provided by Prof. Elizabeth S. Sterner at Lebanon Valley College
Last but far from least, even when you have polymer with high enough molecular weight to have the properties you need for whatever application you have in mind, the chain ends are STILL active. They can still react to give even higher molecular weight, or if you've a mind to, you can react them with some kind of monofunctional terminating group that imparts some property you want in the polymer. You could tie in an antibacterial agent, a flame retardant or a dye molecule to make the polymer colored. Wonderful versatility!
Chain-Growth Mechanism
Whew, the excitement is building- feel it? It's the excitement of knowing what happens at the molecular level rather than at the macroscopic or physical level that we humans function in. So let's move on to the mechanism associated with most polyaddition polymerizations.
The term "chain-growth" refers to how polymer chains are actually formed. With step-growth, all polymers grow slowly more or less at the same time and rate. With chain-growth, polymers are made one chain at a time. That is, whatever's in your reaction vessel is either monomer or high molecular weight polymer. There aren't any oligomers (unless you do things wrong like Neo did) and you could stop the reaction at any time, precipitate the polymeric component and filter it from the left over monomer, and it should be perfectly usable. Take a look at the graph above for the plot dealing with chain growth. The only reason the line doesn't immediately jump up to the maximum value is because it represents an average: lots of low molecular weight monomer and a few high molecular weight polymers.
In actual fact, it's almost impossible to use up all the monomer in this type of polymerization. Why? Simple reason is that as conversion gets high, there's not enough monomers left to find the active chain end to add to it. The rate of polymerization grinds to a halt (so much for whetting your appetite) and you might as well just end it so you can get your polymer out.
Another very important aspect of this type of polymerization is that things happen fast. Chains literally "zip up" as fast as monomer can find an active chain end. Oh, but there can't be very many of those because they react with each other, at least for radical polymerizations.
Let me say that a different way: you only can start so many chains at any given time if you want high molecular weight. Start too many and the ends find each other, couple and they can't react any more. Problem is, the more active ends there are, the faster is conversion, which is desirable from an industrial perspective. But if the reaction goes too fast, things get out of hand: exotherms that vaporize monomer and blow the lid off your reaction vessel.
Things get even more complicated when we talk about "living" polymerizations. Look at that plot in the graph above. It summarizes how molecular weight grows with conversion for polymerizations that have a special set of properties. For this kind, the only polymer chains growing during reaction all start almost at the same time. Unlike radical polymerization (where growing chains terminate continuously while new chains are started, also continuously), this means that all chains grow at the same rate. Thus, molecular weight builds slowly with conversion but not as slowly as with step growth. And here's the important point about living polymerizations: even if all or most of the monomer is used up, the chains can STILL react. They just sit there, waiting patiently like a New York bus rider ready for the next bus, till something happens. That something could be a quenching agent that kills the living end. Or that something could be the addition of more monomer so that polymerization picks right up again. Most important is the fact that the next batch of monomer added could be the same OR different from the first batch. That's how you make "block" copolymers from vinyl monomers, folks.
Confused yet? It's not really all that complicated. Why not make a list or a table with the characteristics and consequences of each type of polymerization. That will help you see key differences and why you do each the way you do so you get usable polymer without blowing anything up. I'd do it for you, but the exercise is good for you (heard that before, have you?). On to ROP.
Ring-opening- Some Combination of the Two
And of course, "ROP" is not some variant of "RIP" but rather "ring-opening polymerization." And here's where things aren't so clear cut. Most ROP's react with some initiator starting the process by opening one ring. The polymer grows only at the active chain end, it grows fast and at some point a side reaction or terminating molecule stops it... or not. Then another initiator starts another chain, and so on: one chain at a time just like for chain-growth. But here's the catch: the propagation step involves the type of intermediate and/or transition state found for step-growth polymerization plus the structure of the functional groups and polymer chain look like those of step-growth polymers.
And to throw another fly into the ointment (really?), some ROP's act just like living polymerizations. They follow the same plot as shown in the graph above, they can make block copolymers or they can be functionalized with desirable terminating groups. Weird and wonderful are the ways of polymers!
And to make things even more complicated, it turns out you can usually make the polymer made via ROP by simple polycondensation using a step-growth mechanism. For example, take the caprolactone monomer in the example above, hydrolyze with water to give you an A-B monomer with an alcohol group at one end and carboxylic acid at the other. Now do the normal polyesterification reaction in which any alcohol can react with any acid to give a backbone ester and you have a step-growth polymer. More complicating is the fact that just looking at this polymer and the one made by chain-growth process wouldn't tell you which is which. Oh, sure, there would be clues in what kind of chain ends are present, but overall, the molecular compositions would be identical as would their properties. May seem confusing, but like I said before, "it's complicated."
So which type of definition should I use, traditional or mechanistic? Depends on what day of the week it is, who you're talking to and what point you're discussing. One kind works fine for some general discussions while the other kind is more appropriate for others that are more detailed and specific. So just like ol' Neo, sink or swim, learn by doing, and practice trial by error. Or put in your own trite phrase here. Time to move on to the next sections where we look at individual families and examples of all three (now actually four) types of polymerizations. We'll delve into why a given polymerization mechanism for a given monomer or set of monomers gives the structures and properties that it does.
Right now, though, it would help you a lot to know what you DO know and more to the point, what you DON’T know. You may not much like taking quizzes, but hey, they’re actually fun! And they really help you understand where you are in the learning process. So let’s take one, ok?
This quiz is about classification schemes for polymers, both traditional and mechanistic. Don't be afraid to guess- your subconscious will often times pop up the right answer even if you're not sure of it: classification evaluation quiz.
And when you're ready to move on, you can begin exploring the details of chain growth polymerizations with all the joys and complexity they offer. In fact, you could pick any of the other topics you'd like to pursue next; just go to the panel on the left at the top and click away...
